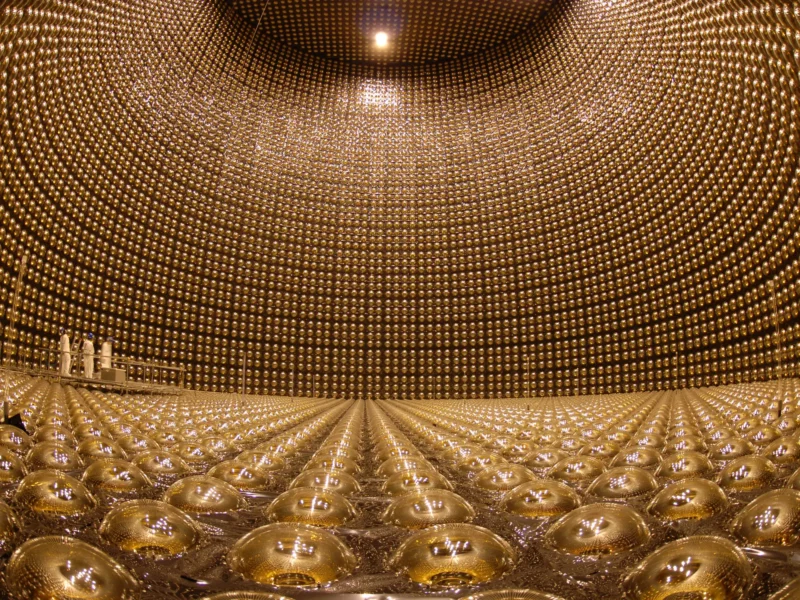
Somehow, neutrinos went from just another random particle to becoming tiny monsters that require multi-billion-dollar facilities to understand. And there’s just enough mystery surrounding them that we feel compelled to build those facilities since neutrinos might just tear apart the entire particle physics community at the seams.
It started out innocently enough. Nobody asked for or predicted the existence of neutrinos, but there they were in our early particle experiments. Occasionally, heavy atomic nuclei spontaneously—and for no good reason—transform themselves, with either a neutron converting into a proton or vice-versa. As a result of this process, known as beta decay, the nucleus also emits an electron or its antimatter partner, the positron.
There was just one small problem: Nothing added up. The electrons never came out of the nucleus with the same energy; it was a little different every time. Some physicists argued that our conceptions of the conservation of energy only held on average, but that didn’t feel so good to say out loud, so others argued that perhaps there was another, hidden particle participating in the transformations. Something, they argued, had to sap energy away from the electron in a random way to explain this.
Eventually, that little particle got a name, the neutrino, an Italian-ish word meaning “little neutral one.” Whatever the neutrino was, it didn’t carry any electric charge and only participated in the weak nuclear force, so we only saw neutrinos at work in radioactive decay processes. But even with the multitude of decays with energies great and small happening all across the Universe every single second, the elusive nature of neutrinos meant we could only occasionally, rarely, weakly see them.
But see them we did (although it took 25 years), and for a while, we could just pretend that nothing was wrong. The neutrino was just another particle the Universe didn’t strictly need to give us but somehow stubbornly insisted on giving us anyway.
And then we discovered there wasn’t just one neutrino but three of them. For reasons the cosmos has yet to divulge to us, it likes to organize its particles into groups of three, known as generations. Take a nice, stable, regular fundamental particle, like an electron or an up or down quark—those particles represent the first generation. The other two generations share the same properties (like spin and electric charge) but have a heavier mass.
For the electron, we have its generational sibling, the muon, which is just like the electron but 200 times heavier, and the tau, which is also just like the electron but 3,500 times heavier (that’s heavier than a proton). For the down quark, we have its siblings, the “strange” and “bottom” quarks. And we call the heavier versions of the up quark the “charm” and “top” quarks. Why does the Universe do this? Why three generations with these masses? As I said, the cosmos has chosen not to reveal that to us (yet).
So there are three generations of neutrinos, named for the kinds of interactions they participate in. Some nuclear reactions involve only the first generation of particles (which are the most common by far), the up and down quarks, and the electrons. Here, electron-neutrinos are involved. When muons play around, muon-neutrinos come out, too. And no points will be awarded for guessing the name of the neutrinos associated with tau particle interactions.
All this is… fine. Aside from the burning mystery of the existence of particle generations in the first place, it would be a bit greedy for one neutrino to participate in all possible reactions. So it has to share the job with two other generations. It seemed odd, but it all worked.
And then we discovered that neutrinos had mass, and the whole thing blew up.
Reader Comments (217)
View comments on forumLoading comments...